IceCube discovers neutrinos from the Milky Way
- News
- Research
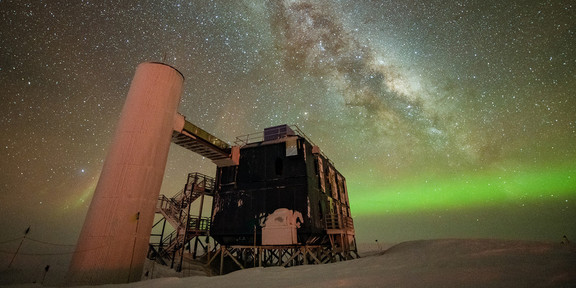
For many people, the sight of our home galaxy, the Milky Way, in the summer from a dark viewpoint is one of the most impressive experiences of nature. Even to the naked eye, the hazy band of light stretching across the sky, the stars interspersed with dark clouds, gives us an impression of the many billions of stars that occupy our cosmic habitat. Although humankind has only been able to solve the structure of our galaxy in modern times, the sight of the Milky Way in visible light has been part of our natural heritage since antiquity. With the IceCube Neutrino Observatory, it has now been possible for the first time to create a picture of the Milky Way with the help of neutrinos – penetrating elementary particles that bear testimony to high-energy processes.
“What is intriguing is the fact that – unlike in the electromagnetic spectrum, that is, light of different wavelengths – in the neutrino regime the distant Universe radiates far beyond our immediate cosmic neighborhood. Therefore, in order to discover our own galaxy, it was necessary for many outstanding researchers across all borders to collaborate,” says Francis Halzen, professor at the University of Wisconsin in Madison, USA, and principal investigator of the IceCube project.
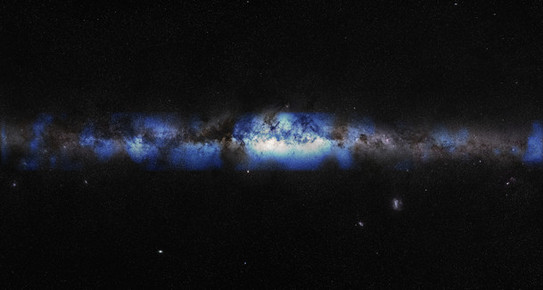
The energy of the neutrinos now detected by IceCube is millions to billions of times greater than that of the steady stream of particles which reaches Earth after nuclear fusion reactions in the Sun’s core. The neutrinos deliver an insight into high-energy particles – cosmic rays that penetrate the space between the stars and constantly rain down on Earth’s atmosphere.
Operated from the Amundsen-Scott South Pole Station, the IceCube detector comprises a cubic kilometer of Antarctic ice, into which over 5,000 light-sensitive sensors have been lowered. Although almost all neutrinos penetrate the matter surrounding us almost unhindered, such a cosmic neutrino does indeed interact now and then in or near the instrumented ice after its long journey through the Universe. Charged elementary particles, for example electrons, are then produced, which trigger short flashes of light in the highly transparent ice, in this way revealing the neutrino and its approximate origin. On the basis of observations of cosmic radiation and high-energy photons – gamma radiation – from the Milky Way, it had already been predicted that neutrinos ought also to be detectable from the band of the Milky Way because they form almost inevitably when high-energy protons and other atomic nuclei interact, for example, with the gas and dust in the space between the stars.
Machine learning facilitates breakthrough
However, it also emerged that the Milky Way is not a particularly copious source of neutrinos. Instead, the scientists faced many major obstacles before the weak signal could be extracted from the overall noise. To overcome these obstacles, the team at Drexel University (USA) set to work on developing analyses that specifically select “cascade events” in the ice, where the energy of the original neutrino is deposited in a relatively compact and roughly spherical region. This approach already led to greater sensitivity for the Milky Way neutrinos they were seeking. This alone, however, was not yet enough for humankind’s first glimpse of our home galaxy on the basis of neutrinos. It was only by applying machine learning methods developed at TU Dortmund University that the breakthrough finally came. These made it far easier to identify the cascades generated by neutrinos and significantly improved the reconstruction of their direction and energy.
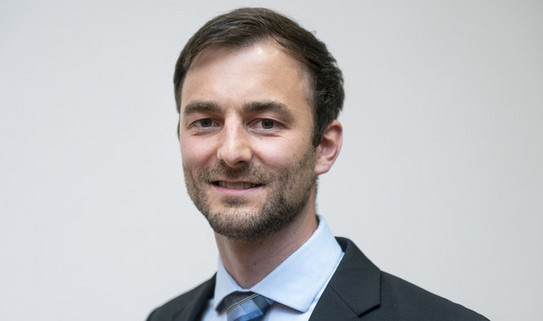
“Developing new methods allowed us to capture more neutrino events, and with better reconstruction of their direction of origin, which effectively led to us being able to increase IceCube’s sensitivity by a factor of three compared to previous searches,” says Mirco Hünnefeld, who as a member of the IceCube Collaboration was one of the lead analysts for this dataset and is completing his doctoral degree at TU Dortmund University.
The dataset used in the study comprised about 60,000 neutrinos from ten years of IceCube observations. That is about 30 times as many events as were used in the selection in an earlier analysis of the galactic plane on the basis of cascade events. The recently discovered neutrino events were compared with previously published prediction maps of regions in the sky from which particularly large numbers of galactic neutrinos were expected.
“The really convincing evidence of the Milky Way as a source of high-energy neutrinos has stood the collaboration’s rigorous internal tests,” says Ignacio Taboada, professor at the Georgia Institute of Technology (USA) and IceCube spokesperson. “Now, the next step is to identify specific neutrino sources within the Milky Way.”
This and other questions will be addressed in follow-up analyses already planned by the IceCube Collaboration. However, it is already clear that the first detection of high-energy neutrinos from the Milky Way affords completely new possibilities for studying the energetic particles in our cosmic environment and constitutes an important step towards understanding the origin of galactic cosmic rays. The enormous capability of state-of-the-art machine learning methods offers great potential for the future and brings further breakthroughs closer within reach. It is only through the cooperation of various institutes that this important step for astronomy and astroparticle physics has been possible.

In Germany, the collaboration comprises ten universities as well as DESY and KIT, two Helmholtz research centers. The further expansion of the IceCube detector and the scientific evaluation of the data collected are primarily funded by the Federal Ministry of Education and Research (ErUM-Pro funding line) and the German Research Foundation. “This long-awaited detection of cosmic ray interactions in the Milky Way is also a wonderful example of what we can achieve if we consistently promote the use of state-of-the-art machine learning methods in basic research,” says Wolfgang Rhode, Professor of Astroparticle Physics at TU Dortmund University, IceCube member and supervisor of Mirco Hünnefeld’s doctoral thesis.